When I was 12 years old, my first PC had 1.5 GB of hard drive storage and I was desperately trying to free up some space to install an old-school, CD-ROM-based, 256-bit, Robin Hood video game, which required around 700MB of storage. Now, 15 years later, tiny solid-state flash drives feature up to 256 GB.
When my dad was a child, mobile phones had not yet been invented and TVs were still black and white. When my dad´s dad was a child, any of this would have seemed like an alien invasion. In contrast, when my child has a child, perhaps she will be living most of her life in a VR-based environment with extensive brain-computer interface integration and the real world might be boring (or uninhabitable).
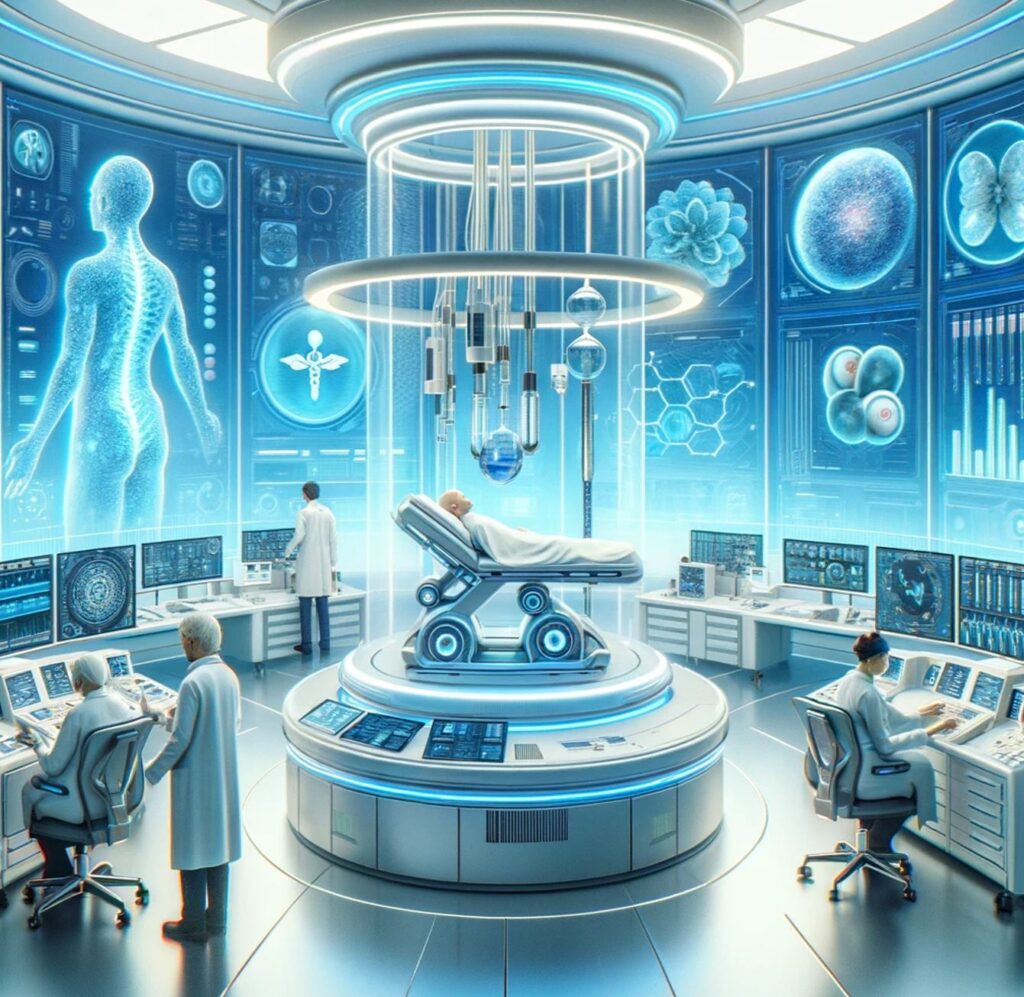
All of this occurred within only a single century, 0.1% of the history of modern humanity, and 1/38.000.000 in the history of life on Earth. Because we are within a moving system, we do not perceive its rate of movement.
Even though there is a vast amount of progress in anti-aging research, until recently, the general public has not been aware of it. And in the coming years, progress will happen even faster – perhaps so fast that we will not even have the time to be astonished. As futurist Ray Kurzweil puts it: “Live long enough, to live forever.” He is referring to people potentially living long enough to reach “longevity escape velocity”.
What follows is a selection of what I think are the most efficient, effective, and promising approaches to increase human lifespan and health span. None of these are uber-optimistic transhumanist sci-fi stuff. Most of these are already in the testing phase in animals (if not humans) and will possibly be available within a few years, or decades at most.
Using the new technologies described shortly, many humans alive today may be able to achieve a longer lifespan than the oldest human being ever recorded (122y) – barring existential catastrophe. Taking into account the warp speed of technological and scientific progress, it is plausible that the first person living for a thousand years has already been born.
New milestones in treating chronic diseases
Living longer largely comes down to how long one can delay the onset of chronic disease. Centenarians don’t seem to live longer once they get a disease, they just seem to take longer to get a disease in the first place. As Dr. Peter Attia says: “The superpower of centenarians is how long they can go before they get the first chink in their armor.”
Three chronic diseases together kill around 80% of people in the civilized world. Thus, any advancement made towards preventing or treating these will lift a major limit on human lifespan in an analogous way that antibiotics or vaccines once did.
Because these “diseases” are highly polyfactorial, there are hundreds of conceivable strategies that could be useful for improving their detection, prevention, or treatment. I will now highlight some avenues I find particularly promising.
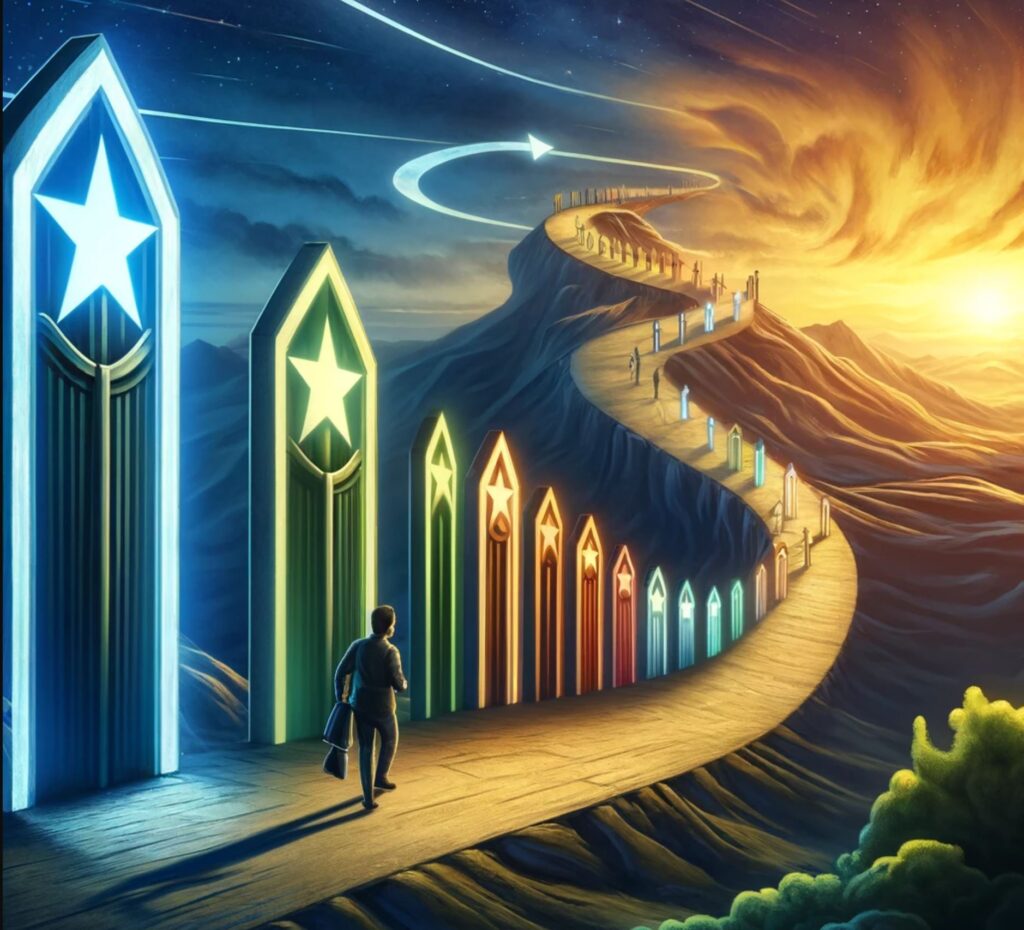
Atherosclerosis
Cardiovascular disease starts early. Putting people on statins when they are 50 is probably not the best way we should go about it. There are many potential ways in which R&D could help. For example:
- Researching therapies that change the way lipoproteins interface with the endothelium
- Using nanotechnology to remove atherosclerotic plaques
- Identifying genetic or endocrine factors of individuals who never get a heart attack despite the presence of strong risk factors, and then targeting these pathways
I discuss the strategies I personally employ to fight atherosclerosis in more detail here.
Neurodegeneration
To some extent, neurodegeneration happens to everyone. However, there are a vast number of things that could potentially be done to prevent or delay it. For example:
- Researching whether mTOR inhibitors (such as rapamycin) help to prevent or at least delay neurodegenerative disorders
- Genetically modifying plants (e.g., corn, soy, sunflower) in a way that they replace 18-C-omega-6-fatty acids with ALA or EPA omega-3 fatty acids
- Treating prediabetics early and aggressively
I discuss my own anti-dementia protocol in more detail here.
Cancer
There are many things that could help us to better prevent, detect, and fight cancers. For example:
- Researching and developing liquid biopsies and employing them on a population-wide level
- Researching and developing immunotherapy to attack cancers based on individual mutations
- Employing more multi-faceted cancer treatment including immune-based therapies and metabolic therapies (in addition to current treatments)
- More widely employing general anti-aging drugs that are known to reduce the incidence of cancer (e.g., SGLT-2-inhibitors, mTOR-inhibitors)
- Counteracting parts of age-related immunosenescence by vaccinating all of humanity against CMV and EBV (two herpes viruses)
Because cancer will presumably be the final enemy, I discuss exciting developments in cancer detection and treatment in more detail here: Exciting New Avenues to Revolutionize Cancer Treatment.
Stem cells
The idea of stem-cell-based intervention has been around for quite a long time. Unfortunately, ethical and legal restrictions have ever since hampered progress in this area. Encouragingly, several potential therapeutic approaches are currently being tested in many laboratories around the world.

The basic idea is to get undifferentiated cells to differentiate into whatever cells we want. This is done by using cocktails of cell-type-specific growth factors to guide cellular differentiation. From replacing cardiac muscle after a heart attack to repopulating degenerated dopaminergic neurons in Parkinson’s disease, the possibilities are endless.
Furthermore, next to being used therapeutically (e.g., to repair organ damage), stem cells can be used to increase the number and size of already existing local stem-cell niches, which can repopulate tissues during repair and maintenance.
The currently tested approaches range from stem cell infusions to implantation of stem cells into an existing tissue structure to induction of residual stem cells already found throughout the body. Time will tell whether stem cells are hype or hope.
Subscribe to the Desmolysium newsletter and get access to three exclusive articles!
Tissue engineering
At the moment, only a limited number of (partial) organ replacement therapies are available, including cochlear implants, knee & hip replacement, and heart valves.
In the near future, various approaches to tissue engineering may be used to replace or restore various damaged tissues, organs, or body parts. For example, let’s create a liver. There are multiple ways to do so:
- We could bombard cultured stem cells with a cocktail of transcription factors that nudge the cells towards differentiating into hepatocytes (the main cell type in liver parenchyma). These can then be implanted into an external synthetic tissue scaffold, which then guides organ structure formation. In this way, one can create a liver ex vivo (outside the body), which could then be transplanted into a human.
- We could gene-edit pigs or other GMO mammals in a way that their cells are not immunogenic to human immune surveillance. We could then harvest the liver and transplant it into a human.
- We could resect the most part of a human liver. We could then use cocktails of liver-specific transcription factors to induce remaining local stem cell niches (or autologous grafted stem cells) to recapitulate the program of morphogenesis creating a new liver in situ.
- We could “print” a human liver using biocompatible materials. This can even be done using cell-based “bio-inks” (basically “liver-tissue-paste”).
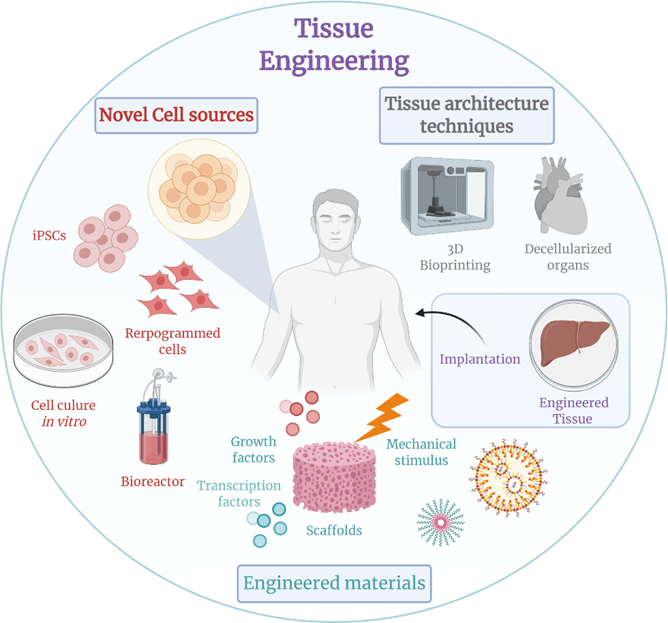
Eventually, due to advances in tissue engineering, most of the human body could in theory be replaced – other than the brain, as the structural and especially functional connectivity of CNS neurons is (likely) impossible to recapitulate.
Resetting the methylation clock (“partial reprogramming”)
As animals age, they accumulate methylations to their DNA in places where they are not supposed to be. This can be measured in the form of the famous (or infamous) “epigenetic clock” (Horvath clock). Some researchers believe that the progressive accumulation of stochastic methylations gives rise to “noisy” gene expression, meaning that cells do not transcribe the right genes at the right times.
However, no one knows whether these changes to the epigenome are causally related to the aging process, or whether they are just a coincidental bystander feature.
“Epigenetic reprogramming” means resetting the transcription status of a cell to a stem cell level (i.e., to an undifferentiated state). By “partially reprogramming” a cell, one can therefore turn back the epigenetic clock, which is thought to be one (key?) aspect of aging.
Using a combination of three to four master transcription factors (the so-called “Yamanaka factors” – OCT4, SOX2, KLF4, MYC) the epigenomic state of a cell can be reset to a more embryonic-like state with much less epigenetic “noise”. This is usually accomplished via gene therapies (e.g., via viral-based vectors) using doxycycline-inducible promoters.
Reprogramming resembles the process of fertilization, during which the epigenome of the parent cells is effectively reset.
These induced pluripotent stem cells derived from aged cells show extended telomeres and improved nuclear and mitochondrial morphology. Furthermore, their transcriptomic profiles seem to be largely restored. Interestingly, after re-differentiation into other cells, many of these beneficial changes tend to remain in the rejuvenated state.
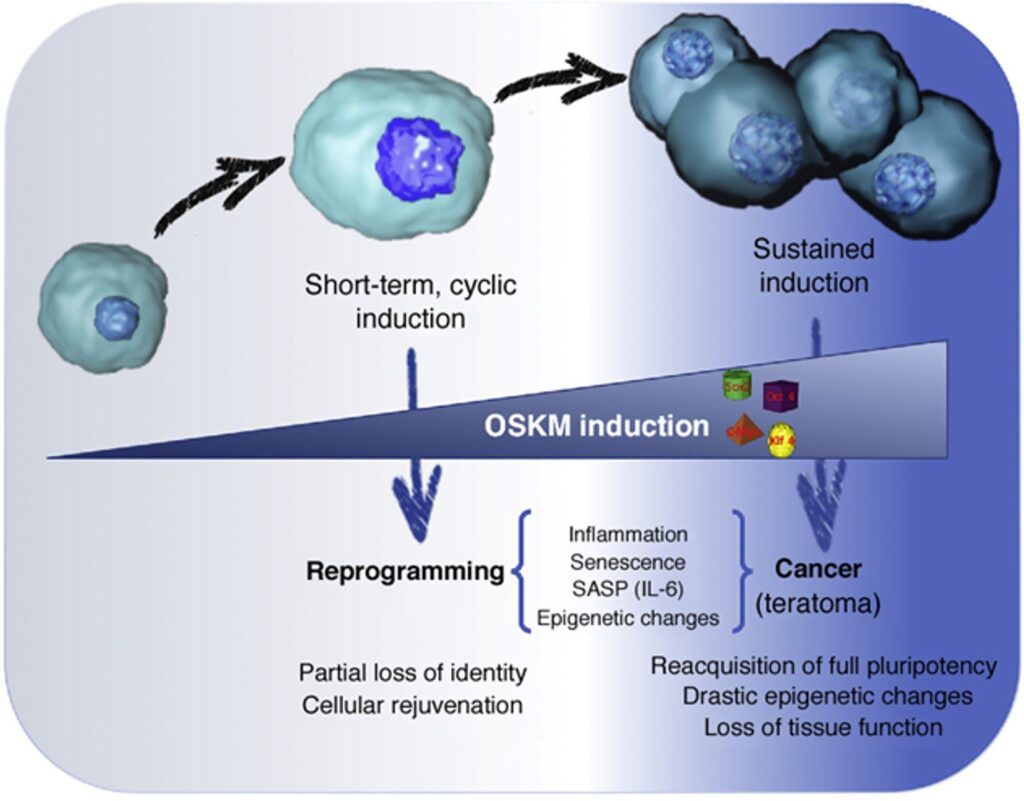
Some people, such as Sam Altman or David Sinclair, are very excited about this process. However, in my opinion, partial reprogramming is mostly useful on a tissue-level scale, particularly suitable for tissues with little cell growth (e.g., spinal cord, inner ear, eye), but not so much on a whole-body scale.
Speculatively, resetting the methylation clock on a body-wide scale may increase cancer risk. Furthermore, it may cause the partial loss of cellular identity possibly leading to noisy cellular behavior and organismal dysfunction.
In sum, partial reprogramming is an exciting possibility but whether restoring a youthful epigenome translates into a youthful phenotype, and therefore holds the key to a prolonged rejuvenated state, is currently not known. However, there are a bunch of laboratories that are currently working on answering this question.
Of note, instead of whole-body reprogramming, a reasonable and probably safe starting point is to partially reprogram lymphocytes ex vivo (i.e., in the laboratory) and then infuse them back into the patient. Having more youthful and “sharper” lymphocytes, which can easily be killed if something goes wrong, may reduce the risk of cancer (due to stronger immune surveillance), age-associated low-level inflammation (“inflammaging”), and infections.
Gene therapy
Longevity is strongly influenced by genetic factors (much more than most people appreciate). In fact, almost anybody who is a centenarian today is a centenarian because of their exceptional genes – having a healthy lifestyle is helpful but is far from sufficient by itself.
Some of these genes have been identified. There are hundreds of them, some more important than others. By using gene therapy approaches it is possible to either introduce mRNAs for direct protein expression (e.g., by using liposomes), DNA to be shuttled to the nucleus for transcription to mRNA (e.g., by using viral vectors), or even modify our genome (e.g., by using CRISPR-based technologies).
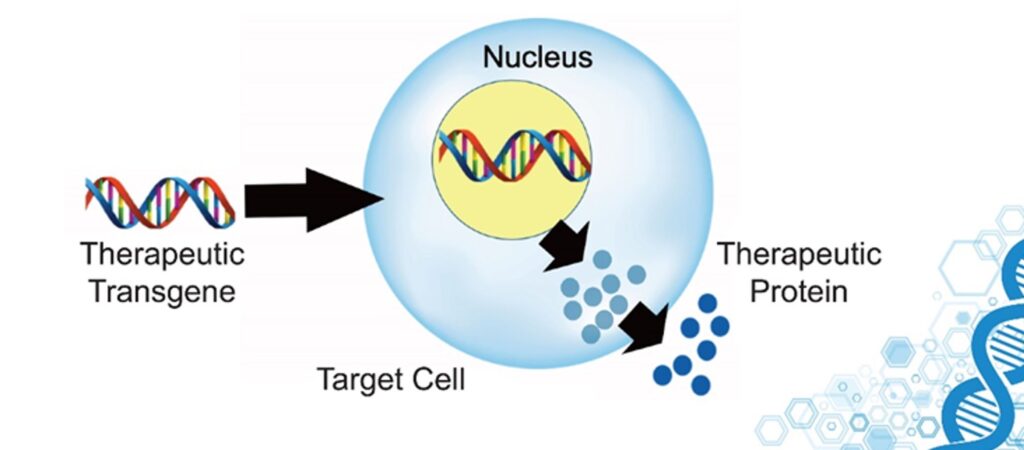
For example, we could:
- Edit, repair, or replace deleterious alleles (e.g., APOE4, BCRA1, APP, etc.)
- Upregulate the expression of longevity genes by modifying the promoter regions or regulating splicing sites (e.g., FOXO3, p53, antioxidant systems, DNA-repair proteins, chaperones, heat shock proteins, autophagy-associated proteins, etc.)
- Downregulate the expression of pro-aging genes (e.g., telomere eroding factors, mTOR and associated proteins, growth factors and proteins involved in their signaling cascade, proteins associated with the PI3K-RAS-MAPK-pathway, senescence-specific proteins, pro-cancer genes, methylation enzymes, etc.)
- Knock in completely new genes (e.g., longevity genes from other species given that they are non-immunogenic in H. sapiens)
Telomerase induction
One gene that deserves special mention is telomerase. Telomerase is an enzyme that lengthens telomeres. With every cellular replication, telomeres progressively shorten. Sufficient telomere erosion is one of the many ways to induce cellular senescence, preventing cells from dividing any further.
In human fibroblasts, this limit is capped at about 75 cell divisions (“Hayflick limit”). Interestingly, the Hayflick limit of mice fibroblasts is about 15, whereas some long-lived giant tortoises have Hayflick limits of about 150 cell divisions.
The Hayflick limit is partially dependent on telomere length, which is partially dependent on telomerase activity. Telomerase is highly active in developing cells and stem cells, allowing them to proliferate without losing the ability to do so (potentially indefinitely).
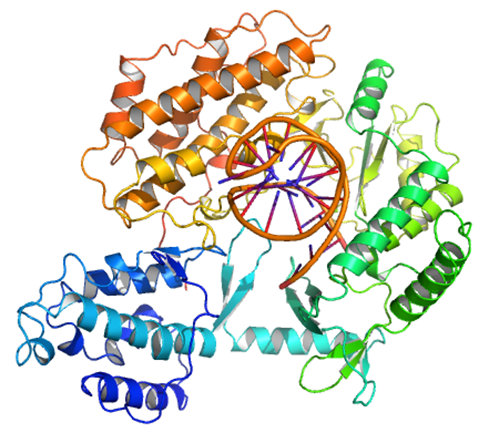
However, telomerase is a double-edged sword. The more often a cell has divided, the higher the mutation load because at every cellular division, there are going to be a couple of stochastic replication errors. Preventing these cells from dividing prevents potentially cancerous cells from spreading.
Therefore, increasing telomerase activity may help carcinogenesis. On the one hand, telomere erosion (due to excessive cellular replication + lack of sufficient telomerase activity), stops cells from dividing any further, inducing cellular senescence (why this is “bad” is discussed at length here).
Telomerase activation via pharmaceutical drugs or overexpression via gene therapy could be a way to prevent this telomere-erosion-induced pathway to senescence (there are other pathways to senescence also). The downside is that it comes with a potentially increased risk of cancer. However, via advances in cancer screening technologies (especially liquid biopsies -discussed shortly), potential cancers may be found and taken out early.
Parabiosis
Whenever the circulatory systems of a young and an old animal are joined (parabiosis), some rejuvenation happens in the old animal (including the extension of lifespan). This is the reason behind Bryan Johnson using his son as a “blood boy”.
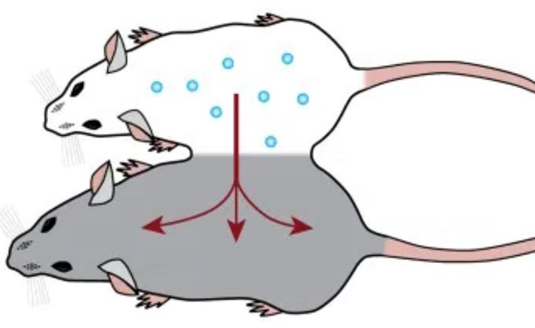
This could be due to either stem cells from the young animal repopulating the more degenerate tissue of the old animal, or it could be due to the transferring of some as of yet mostly unidentified blood-borne factors (e.g., specific growth factors). In the latter case, we could identify these factors, clone them, and use them therapeutically the way we use insulin or growth hormone.
Senolytics
Senescent cells, as holds for every other cell, are kept alive by the continuous signaling of certain growth factors. However, depending on the tissue and cell type, these growth factors (and their receptors) differ. By antagonizing (blocking) the growth factors that are important for the survival of a specific type of senescent cells, some of these “zombie cells” will undergo apoptosis (controlled cell death) much earlier than they would otherwise.
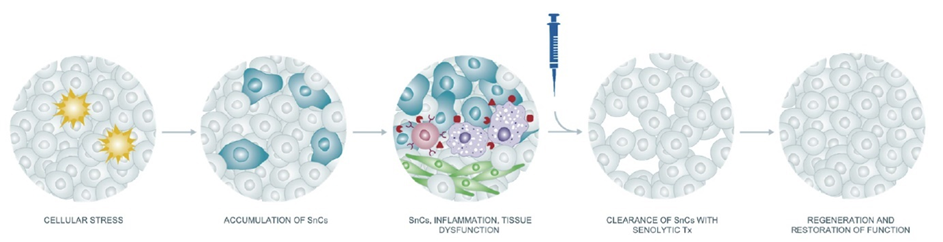
For example, the senolytic agent dasatinib (I discuss my experience with it here) targets certain receptor tyrosine kinases more than others and is therefore rather “tissue-selective” in its senolytic action (e.g., it kills senescent cells in adipose tissue but not heart tissue).
There are multiple other senolytic agents currently in pharmaceutical pipelines, most of them originally developed as cancer drugs. By combining a variety of different senolytics in specific combinations, one could repeatedly clear specific tissues (and perhaps even the whole body) of senescent cells.
After a short course of “hit-and-run” treatment (e.g., for a couple of days), this could be followed by a short course of growth hormone or IGF-1 treatment in order to induce local stem cells to proliferate and to repopulate the now vacant cellular niches.
Subscribe to the Desmolysium newsletter and get access to three exclusive articles!
Nanotechnology
There is a lot of hype around nanotechnological innovations for medical purposes. Theoretically, products of nanotechnology might be used for waste removal (e.g., metals, plaques, metabolic aggregates), as antiviral “robots”, or for carrying out specific functions at a tissue level.
In my non-expert opinion, despite Moore’s law, there is no nanotechnology close to being ready within the next 10-20 years, and true medical nanotech will probably remain sci-fi for a long time.

Furthermore, even with the most incredible future nanotechnologies, in my opinion, it is utopian that our cellular repair and housekeeping machinery will ever be perfect. For example, it will be impossible to completely prevent spontaneous mutations from happening.
Mind uploading
The more I learned about neuroscience, the more I realized that “mind-uploading” is bullshit propagated by tech people without any understanding of biology. Proponents believe that neurons can be replaced with simple on-off nodes in a neural net by making these nodes highly dynamic and capable of adapting and rewiring.
However, neurons are living cells influenced by paracrine & autocrine & endocrine factors in addition to the local non-neural environment (e.g., glial cells, electrolytes, gases). Furthermore, every single neuron is its own cellular micro-universe. All of this makes neuronal activity infinitely complex and not just a product of summing up various inputs to produce an output.
Some people want to go around this problem by crudely simulating neurons. However, approximating real-life neuronal activity would presumably entail simulating the stochastic and probabilistic nature of quantum mechanics at least to some extent.
Furthermore, even if one could do all that, the activity of our nervous system depends on non-stop input from sensory cells transducing the external and internal environment. Everything my nervous system does at any given point depends to a large degree on this continuous input (next to some spontaneous neural activity).
If one were to perfectly simulate the nervous system, where would this continuous sensory input come from?
This sensory input does not just depend on our “five” senses, but dozens of sensory modalities and thousands of sensory feedback loops that never even make their way up to the neocortex and thus into consciousness.
For example, the millions of chemo- and mechanoreceptors all over the body continuously transmit data to the brain stem, which in turn modulates CNS activity in a specific way and imperceptibly guides my thinking, feeling, and acting. In my opinion, both problems (simulating neurons; simulating input) are insurmountable. Not just technically.
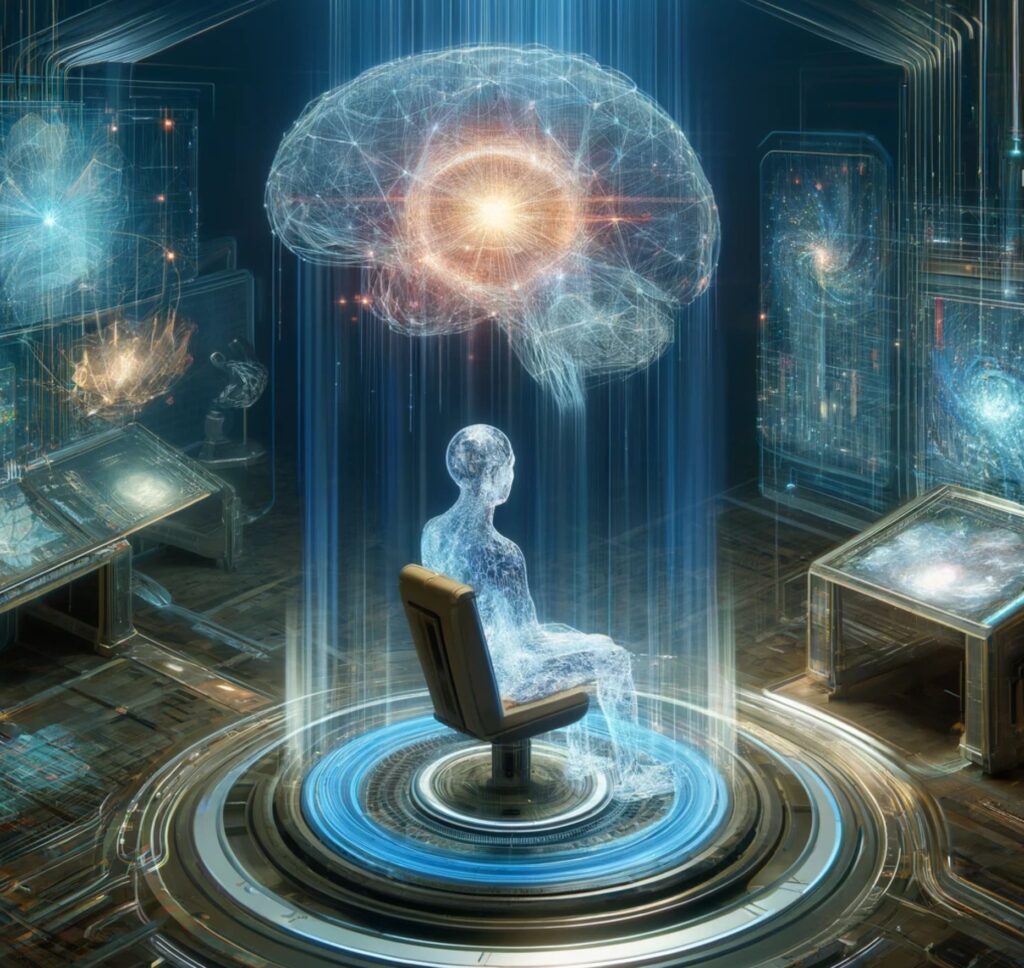
Off-topic note on non-biological consciousness
While mind-uploading is, in my opinion, utopian, it is indeed possible that our technological children will survive and have their own “minds”.
It is more likely than not that the right kinds of computations are sufficient for the emergence of consciousness. Given that “substrate independence” is true, consciousness is just an emergent property of complexly arranged matter (e.g., as in our brains).
Consequently, when implemented with sufficient complexity, the “right” processing architecture might cause consciousness to arise spontaneously. If this architecture were modeled after the human brain, it would probably be characterized by distributed signal representation, large-scale signal integration, and swift feedback and feedforward loops.
Given that, in theory, this is not limited to biological systems, consciousness might spontaneously emerge as a byproduct in artificial agents (including software) that have a suitable (neuro-inspired?) architectural complexity (or anything functionally equivalent) – possibly including noise-driven assemblies resembling spontaneous cortical activity.
Sources & further information
- Scientific article: Stem cells: past, present, and future
- Scientific article: The Effects of Parabiosis on Aging and Age-Related Diseases
- Scientific article: In Vivo Amelioration of Age-Associated Hallmarks by Partial Reprogramming
Disclaimer
The content available on this website is based on the author’s individual research, opinions, and personal experiences. It is intended solely for informational and entertainment purposes and does not constitute medical advice. The author does not endorse the use of supplements, pharmaceutical drugs, or hormones without the direct oversight of a qualified physician. People should never disregard professional medical advice or delay in seeking it because of something they have read on the internet.